
If both the frequency separation, δf, and Q m are maximized, a larger bandwidth-efficiency product is possible,Īs well as high bandwidth and efficiency, the radiated signal magnitude should be maximized. Because an active transmitter is not a linear time-invariant system, the Bode-Fano limit does not constrain the bandwidth.
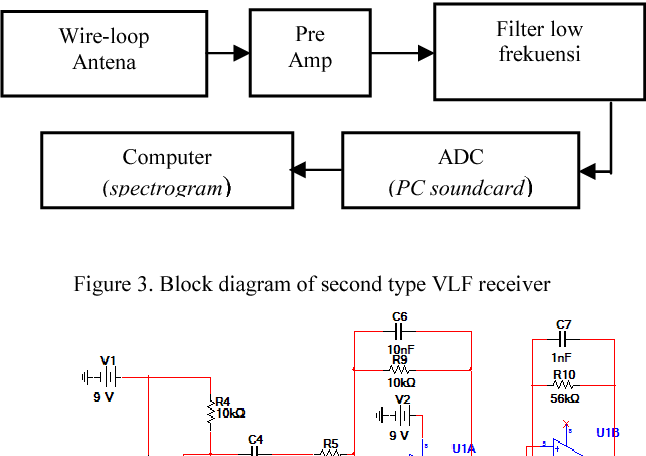
In a frequency shift keying (FSK) modulation scheme, the carrier and hop frequencies each correspond to a different resonant frequency which changes at the FSK rate. DAM enables operation at a frequency outside the fractional bandwidth of the passive antenna. In one embodiment, the resonant frequency is actively shifted coincident with changes in the input drive frequency. This implies that electrically small, low-loss transmitters are also low bandwidth and therefore, by the Shannon–Hartley theorem, have limited data bitrates 20.ĭirect antenna modulation (DAM) decouples bandwidth from Q t 21, 22, 23, 24, 25, 26, 27. Passive, high Q transmitters have small fractional bandwidths,įor a passive antenna, this relationship simplifies to Bη = 1/( \(\sqrt 2\) Q A,min) which is a function of Q A,min, itself a function of the antenna electrical size. An advantage of strain-based antennas is acoustic resonance in a device with physical dimensions much less than the electromagnetic wavelength, potentially removing the need for large, external impedance-matching elements. Radiation has been measured from vibrating quartz resonators 14, 15, 16 and piezo-magnetic or multiferroic antennas have both improved ESAs 2, 3, 17. A potential solution is acoustically resonant transmitters 3, 13. Active non-Foster matching networks, while shown to improve system bandwidth, are unwieldy at high voltages 10, 11, 12. The size and loss of this matching network, even assuming a Q of 1000, greatly exceed the antenna itself, making this technique non-viable. To impedance match to a 10-cm long, 10 mA m rms, 35.5 kHz antenna, a 10.5 H, 125 kV inductor is needed. Consider an electric dipole antenna made up of a copper wire normal to a ground plane. Therefore, to have a measurable increase of efficiency, Q m must be very large.īulky impedance matching networks compound antenna inefficiency. Assuming a wavenumber-length product of 7.5 × 10 −5, the minimum Q A 7, 8, 9 is between 3 × 10 12 and 3 × 10 13 (see methods). If Q m is much less than Q A, then Q t is approximately equal to Q m and therefore, to maximize the transmitter efficiency, η = Q m/ Q A, Q m must be maximized. In a lossy antenna, the total Q is Q t = 1/(1/ Q m + 1/ Q A) where Q m encompasses all non-radiation losses within the antenna system. Although demonstrated at VLF, this concept straightforwardly scales to other frequency bands.Ī significant challenge for ESAs with a wavenumber-length product much <1 is a high radiation Q, Q A, which considerably limits the radiation efficiency 5, 6. These are our fundamental advancements: achieving an exceptionally high system Q with no external impedance matching network and an effective fractional bandwidth beyond the passive Bode-Fano limit 4. Therefore, high total Q (low loss) no longer constrains the system bandwidth.


Utilizing piezoelectricity as the radiating element allows us to dynamically shift the transmitter resonant frequency. Traditionally, a disadvantage of passive high-Q antennas was low bandwidth. These transmitters can resonate in a very small footprint while exhibiting low losses 2, 3. We show that a strain-based, piezoelectric transmitter can overcome many of the fundamental limitations of conventional electrically small antennas (ESA). Conventional transmitter techniques are inadequate due to large size and high loss. Particularly attractive, very low frequency (VLF) signals attenuate <6 dB/1000 km within the Earth-ionosphere waveguide and can penetrate tens of meters into seawater or dirt.
#Schematic vlf receiver portable#
The prevalence of human portable or autonomous vehicle platforms has significantly increased the demand for small, efficient transmitters 1.
